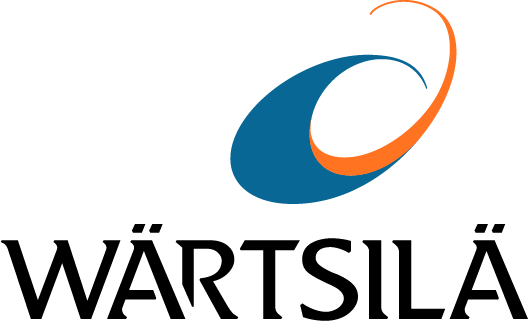
A fuel cell power pack consists of a fuel and gas processing system (the balance of plant), and a stack of fuel cells that convert the chemical energy of the fuel to electric power through electrochemical reactions. The process can be described similar to that of a battery, with electrochemical reactions occurring at the interface between the anode or cathode and the electrolyte membrane but with continuous fuel and air supplies.
The main drivers for developing maritime fuel cell technology are reduction in fuel consumption and lower impact (both local and global) on environment of ships’ emissions to air. Additional benefits include insignificant noise and vibration levels, as well as lower maintenance requirements compared to traditional combustion engines. Key challenges include the demand for clean, low carbon fuel and the need to decrease investment costs, improve service lifetime, and reduce the current size and weight of fuel cell installations.
Different fuel cell types are available and can be characterized by the materials used in the membrane. Molten Carbonate Fuel Cell (MCFC) and Solid Oxide Fuel Cell (SOFC) technologies are high-temperature fuel cells that are flexible as regard to choice of fuel: methanol, ethanol, natural gas, biogas, and hydrogen are most commonly used. MCFC is the more mature of these two technologies, while SOFC is considered to have the greatest potential in terms of efficiency and power density.
An electric stack efficiency of 50-55% has been obtained from both MCFC and SOFC installations, and when internal consumption is included this is lowered to 45-50%. High operating temperatures lead to high exhaust temperatures (400-800°C) that, together with a large volume flux of exhaust, yield a significant potential for heat recovery. The fuel to electric efficiency can be increased to 55-60% for MCFC plants and to above 60% for SOFC plants when heat recovery is included.
A complex balance of plant to handle fuel and air treatment is required for both technologies, as a result larger units are preferred. MCFC units generally have one fuel cell stack of bout 200–500kW, while an SOFC unit is built from several smaller stacks of 1-20kW each.
The SOFC units can be built to be significantly more compact than MCFC units, but the complete power packs remain large in volume compared with diesel generators. Hightemperature fuel cells must operate at stable temperatures, and therefore have low tolerance to rapid load changes. In general, these fuel cell types can only be justified in applications where power and heat demands are high and stable.
A methanol-fuelled marine SOFC plant of 20kW was tested on board the car carrier Undine in 2010. The largest marine fuel cell installation to date is the 330kW MCFC, installed on board the platform supply vessel VIKING LADY designed by Wärtsilä Ship Design. The LNG-powered ship was delivered for operation on the North Sea in April 2009, and, in September of the same year, the 330kW MCFC power pack developed by MTU in Germany was installed. The fuel cell was connected to the main switchboard for the first time in December 2009. After initial testing, VIKING LADY became the first vessel to obtain the class notation FC-Safety, as described in the DNV Rules (DNV, 2008).
The fuel cell stack, together with the required balance of plant, is located in a large, purpose-built container (13 x 5 x 4.4m). Project-specific electrical components (transformers, converters and DC bus) designed to protect the fuel cell from potentially harmful disturbances on the power grid, are situated in a standard 20-ft container. The total weight of the containers is 110t, but both weight and volume could be significantly reduced in future fully integrated systems.
During its first year in operation, the fuel cell stack showed no signs of degradation, indicating that the measures taken to protect the fuel cells were appropriate. The stack was protected against electric disturbances. In addition, ship movements, hull vibrations, and air salinity were also taken into consideration when designing the fuel cell stack and its container and support systems. In January 2012, the fuel cell was cooled down and conserved for future demonstration projects. A total of 18,500 operating hours were logged without signs of severe performance degradation. Approximately half of the time logged was in idling mode.
Fully loaded, the fuel cells produced electricity at a measured electric efficiency of 52.1% based on the lower heating value of the LNG. Although exact measurements of gas to grid efficiency were not possible for the current system setup, this was estimated to be 48.5% including internal consumption, and 44.5% when DC/AC conversion was also accounted for. A heat exchanger that produced warm water from the fuel cell exhaust was tested, with about 80kW heat recovered. This increased the overall fuel efficiency to slightly above 55%. With optimal system integration, there is the potential for increasing the electrical efficiency to close to 50%, and the fuel efficiency up to 60%.
Although the cost, weight, and volume of the test installation were high, the feasibility of installing and operating a fuel cell power pack in a marine environment was successfully demonstrated. In future marine MCFC designs more focus should be directed towards thermal integration, utilizing the high quality exhaust heat, and on including some form of energy storage to allow for stable load conditions for the fuel cell.
Further reading: DNV paper “Fuel cells for ships”