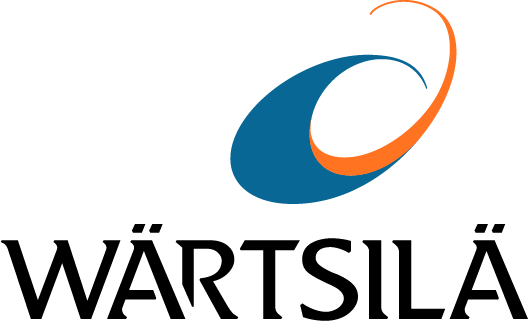
Our modern world runs on electricity, powering our society, driving our economy, and empowering our lives. To make all this possible, today’s electricity grids must respond quickly to the changes in the demand and supply of electricity.
Renewable energy, however, is intermittent. That’s why we need energy storage and flexible power generation to respond to renewables’ variable electricity output, ensuring a stable and reliable power supply. Without this balancing flexibility, we’d suffer from outages and blackouts, putting our lives on hold whenever the sun isn’t shining, or the wind stops blowing.
Every second counts in the pursuit of achieving a 100% renewable energy future. By embracing flexible power generation, we keep the grid stable while accelerating the energy transition and mitigating climate change.
With that in mind, let’s take a look at two competing power generation technologies — reciprocating internal combustion engines and aeroderivative gas turbines. Here are three things you need to know about engines and aeros to understand the best way the world can move towards a future powered mainly by renewable energy.
Simply put, flexibility refers to a power plant’s ability to quickly and easily start, stop, and adjust its output according to the electrical grid’s demand. Let’s break down a few key factors that contribute to flexibility in power generation. For more details and figures, watch our “Shaping the future of power generation with flexible engine power plants” video, which demonstrates the flexibility of engines using real data from South Australia’s energy market.
First, let’s consider the start-up time, which is critical during unexpected drops in supply or sudden surges in demand. Engine power plants can start up and reach full load within a couple of minutes. In comparison, gas turbine plants take about five to eight minutes to reach full load.
Another factor to keep in mind is the minimum up and downtime, which is the minimum amount of time a power plant needs to start or stop generating electricity. Engines have no minimum up and downtime, meaning they can start or stop very rapidly, as needed. Gas turbines, on the other hand, have minimum up and downtimes of at least 10 and 30 minutes. A slow dispatch from aeros can cause power swings detrimental to the grid.
The minimum stable load is the lowest power output a power plant can operate at, and engine power plants have a minimum output of only 10%. Gas turbines must have a minimum output of 40–50% or they will go over the limits of their air pollution permits. This means that they can help keep the grid stable even when there isn’t much demand for electricity, as well as seamlessly adapt to the unreliable output of solar and wind power, which depend on the whims of the weather.
Next, let’s look at the ramp rate, the rate at which a power plant can adjust its output. Rapid ramp rates are important for balancing renewable energy sources. In this regard, both engines and aeros outperform traditional and inflexible power plants. That being said, we specifically designed our engine power plants to ramp their output by a large amount in a very short time, providing unrivalled flexibility.
Finally, let’s think about net efficiency, which is how well a power plant turns energy into electricity. Net efficiency matters for power flexibility because it enables cost-efficient power generation and helps reduce fuel costs. Whereas engine power plants can convert nearly 48% of their fuel to electricity when running at full capacity, gas turbine power plants can only convert 40%.
Engine power plants are usually modular, consisting of a fleet of engines. This modularity allows them to turn on as many or few engines as needed, all the while maintaining high net efficiency. Aeroderivative gas turbine power plants tend to instead be larger and made up of a few gas turbines. This limits their load range, making them less flexible and lowering their net efficiency when running at partial load.
Power plant owners want to optimise profitability by minimising costs and maximising revenue. In the context of variable renewable energy integration, flexible power plants designed with the intermittency of renewables in mind can achieve this goal profitably.
While engines can start and stop an unlimited number of times a day and continuously ramp up and down the output without running up additional costs, the key components of aeros have a cycle limit. And because these include not only starts and stops, but also ramps and trips, you will inevitably accumulate cycles quickly. As a result, at 10,000 cycles, major overhaul of turbine internals is necessary, which increases variable maintenance costs.
In modern energy markets with a high share of renewable energy, where the electricity from generators is dispatched to the grid in five-minute intervals, flexible power generation is not only a must to ensure grid stability but also opens up profitable opportunities.
As we get closer to 100% renewables, flexible engine power plants will be more in demand precisely because they can be relied on to cost-efficiently generate the electricity needed. By swiftly adjusting their output during peak electricity prices, flexible engine power plants can maximise revenues and profits.
However, everything from site conditions (e.g., ambient temperature, humidity, altitude) to operation modes (e.g., running at partial load) to the number of starts and ramping impact the net efficiency and output, not to mention the operating, maintenance, and fuel costs.
Across a range of real-world conditions, engine power plants consistently maintain a higher net efficiency than gas turbine power plants, enabling a reduction in fuel consumption by as much as 28%. The higher the fuel costs, the more important net efficiency is for the bottom line.
In hot and humid environments, the output and net efficiency of aeros is reduced. Granted, demineralised water and inlet air chilling can be used to reduce derating, but this reduces efficiency and can be costly because inlet air chilling consumes a lot of electricity, and aeros consume a lot of water, eroding profits. By reducing net efficiency and output, derating has a negative impact on the revenue of power plants on hot and humid summer days.
Engine power plants are generally more profitable than gas turbine power plants due to their lower variable costs and their ability to respond quickly to changes in electricity demand, without needs for additional maintenance. The truth is that gas turbine power plants are not a good fit for the short-duration cycling needed to balance solar and wind output.
The fuel flexibility of Wärtsilä engines makes them future-proof as we are phasing out fossil fuels and moving forward towards 100% renewable energy. By choosing fuel flexible engines, power producers can minimize the risk of investing in assets that may become unprofitable or obsolete.
We designed our engine power plants to operate on various gaseous and liquid fuels. The engines can run on 100% synthetic, carbon neutral methane and hydrogen/natural gas blends with up to 25% hydrogen. Engines for many hydrogen-based, carbon neutral fuels are under development to enable reliable as well as sustainable power generation.
Engines can switch fuels instantly without reducing load or compromising power plant availability. Aeros can only use either natural gas or light fuel oil. Gas turbine power plants must go offline to switch from natural gas to fuel oil as they are not designed to run on liquid fuels for extended periods.
The importance of true flexibility in power generation cannot be overstated. As we’ve seen, flexible engine power plants hold the key to seamlessly integrating renewables into the grid while maintaining its stability and reliability.
Engines play a critical role in reducing the risk of devastating grid outages and blackouts due to their proven ability to respond quickly to changes in electricity demand and supply.
Because of their flexibility and efficiency, they can balance the grid profitably, making them a good investment for power producers. And their fuel flexibility makes them future-proof.
All things considered, engine power plants are preferable thanks to their superior flexibility, profitability, and future-proof nature. With engine power plants at the forefront of the energy transition, we can confidently move towards a future in which 100% renewable energy is used to meet our growing electricity demands.
These technologies differ in many aspects.
Find out how in the detailed technical comparison articles.
1. Combustion engine vs. Aeroderivative gas turbine: Introduction
2. Combustion engine vs. Aeroderivative gas turbine: Executive summary
3. Combustion engine vs. Aeroderivative gas turbine: Six elements of dispatching
4. Combustion engine vs. Aeroderivative gas turbine: The advantages of modularity
5. Combustion engine vs. Aeroderivative gas turbine: Part-load efficiency
6. Combustion engine vs. Aeroderivative gas turbine: Pulse load efficiency
7. Combustion engine vs. Aeroderivative gas turbine: Derating due to ambient temperature
8. Combustion engine vs. Aeroderivative gas turbine: Fuel flexibility
9. Combustion engine vs. Aeroderivative gas turbine: Water consumption
10. Combustion engine vs. Aeroderivative gas turbine: Greenhouse gas emissions